I seem to always begin writing my posts saying that is has been a very busy couple of weeks and that it is only going to get busier. Well, I can honestly say, that it is definitely true now because my PhD defense date has been officially booked for August 3rd!!! This is now a reality check for me. Now, I have to submit a draft of my thesis to my examining committee (which has been selected, thank you Dr. Catherine Neish!) at the latest June 22nd. This is because a PhD thesis at the University of Western Ontario needs to be submitted at least 6 weeks before your defense date. It looks like I will be going through the final grind during the next month; the final hill before the end of the marathon (oh it has definitely been a marathon, and COVID-19 threw in some weighted running shoes). Anyhow, let us get right into the topic of this post, radar polarimetry!
Radar Polarimetry Workshop
From May 10th to 14th, I virtually participated in the 6th European Space Agency (ESA) Advanced Course on Radar Polarimetry. This ESA course is offered every two years and lasts for 5-days, teaching geoscientists about the basic concepts, advanced concepts, and applications of radar polarimetry. The aim of the course is to train the next generation of earth observations scientists to utilize dual and quad-polarimetric radar data. ESA encourages post-graduate students, PhD students/candidates, post-doctoral scientists, and research scientists or engineers from European countries and Canada interested in radar polarimetry and earth observation to register for the course. Normally, the course is held in Italy (city changes every year; 4th annual was held at ESA ESRIN Rome and the 5th was held in Frascati), but unfortunately due to COVID-19, I had to attend the course virtually. Ironically, I probably would not have been able to register for the course if it was not for COVID-19 because I don't think I could have afforded to go over to Italy for about a week.
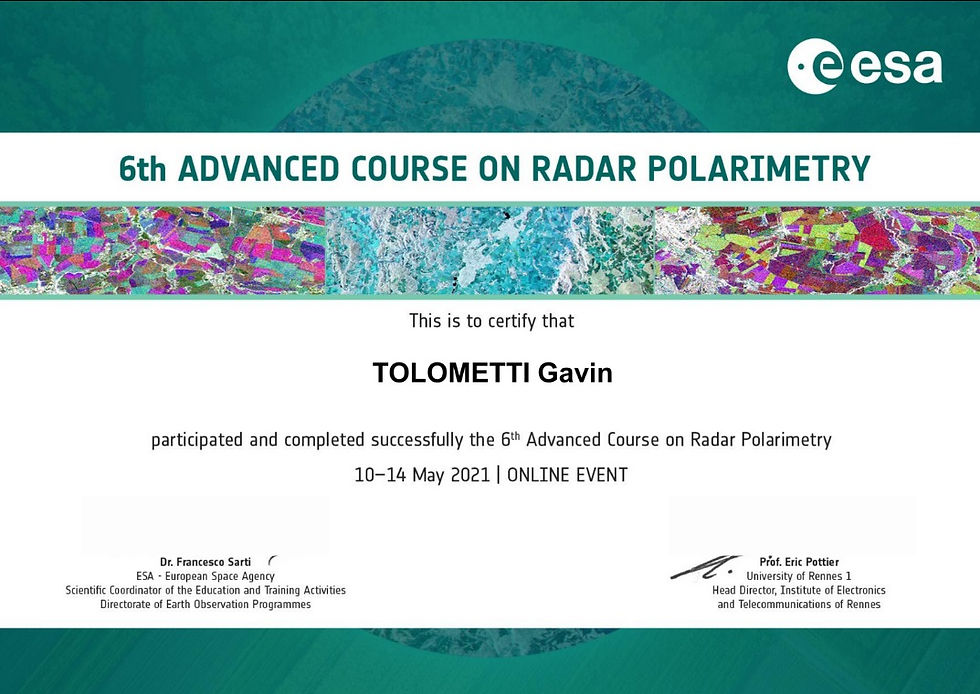
Why Did I Take the Course?
If you have read some of my previous research blog posts you will know that radar polarimetry (specifically synthetic aperture radar) is heavily incorporated into my PhD thesis. You are probably wondering then, "Gavin, why would you take a course on radar polarimetry if you have spent almost 5 years using it to study lava flows?". The short answer is, there is still a lot for me to learn about radar polarimetry and even though I have used it in my studies for almost 5 years I don't consider myself an expert, or even an intermediate user. I wanted to learn more about radar polarimetry and its applications in earth observation (EO).
What Did I Hope To Learn From The Course?
I hoped to learn new methods for speckle noise filtering and processing synthetic aperture radar data. I have gotten accustomed to following methods used in radar planetary science papers, which have helped me immensely during my PhD. However, I knew there was so much more we could do with radar in planetary science. I hoped to take the course and leave with more knowledge on understanding how noise in polarimetric radar data is removed and data is smoothed without loss of surface features, and learn new ways to present and interpret backscatter and polarimetry data.
I will summarize what I did take away from the course at the end of this post.
I am Interested in Radar and EO, Where Can I Register For The Course?
As I mentioned previously, the course is held every two years, unlike other ESA workshops that are held annually. You can find all of the information about the course on the ESA website: ESA Polarimetry Course 2021. They have not released any information for the 2023 course yet - they probably will not release anything until mid-2022 - however, the 2021 link has all of the information you need to understand the aim and structure of the course.
Structure Of The Course: Early Morning Theory Lectures
I probably should mention this, since the course is organized by ESA, the time zone that was set for the program was scheduled to follow Central European Time. For everyone in Europe, it was an 8 am start to about 4:30-5 pm finish. For me, well, a 2 am start to a 10:30-11 am finish. Normally, I would bite the bullet and take an early nap before the course starts and power through the day. However, I have major PhD thesis deadlines to meet by the end of this month and didn't want to through off my sleep schedule. I emailed the course organizers and they said I could asynchronously cover the first set of lectures. This allowed me to sleep until at least 4:30 am. On the course program, we had a scheduled lunch break from 12 pm to 1:30 pm CET (so 6 am to 7:30 am for me). During that time I caught up on the slides I missed during the first 2 hours of the course. I may have not been up for all of the lectures but I was able to stay on top of everything so I was not confused when we walked through the practical's.
Breaking down the lectures in the course, we covered five main topics:
Introduction and Basic Concepts of Synthetic Aperture Radar (SAR)
SAR Polarimetry: Basic & Advanced Concepts and Applications
PolSAR Applications: Theory
Principle and Basics of In-SAR
Understanding 3D SAR Tomography
We would start off each day with a series of lectures to provide us with the theory and basic background knowledge on radar polarimetry concepts, SAR, In-SAR, and Tomo-PolSAR. After the lectures, we would run through a practical assignment, which was mainly an opportunity to visually understand the theory we were taught during the lectures. Now, for the sake of time, typing, and your sanity, I won't be able to cover the entire course in explicit detail in this post. In this post, I will be providing you all with a summary of what SAR data is and how it is used to analyze the surface of Earth.
Basic and Advanced Concepts of SAR
The first two days of the course focused on understanding how we use polarimetric radar to measure the intensity and polarization of scattered radar signals, understand the speckle noise effect and the different filtering models used to clean SAR data, and the EO applications of SAR. We began by covering the basics, such as what is SAR?
SAR is a 2-D representation of a planetary surface (see image 1 below), constructed using data from transmitted and received radar pulses. The remote sensing technique uses microwave EM signals to measure the physical and electric properties of surfaces, in particular, roughness and dielectric constants. It is an active remote sensing technique, emitting its own signal from an instrument instead of relying on an external light source to reflect off of a surface (passive remote sensing). SAR measures the amplitude and phase of microwaves that are emitted and scattered off of a surface (see image 2 below). The amplitude is dependent on the dielectric and geometric properties of a target (changes based off electrical properties and surface roughness), and the phase is dependent on the sensor-target distance and target properties.
Image 1. Animation of the dual-polarization Sentinel-1 satellite; an example of polarimetric radar.
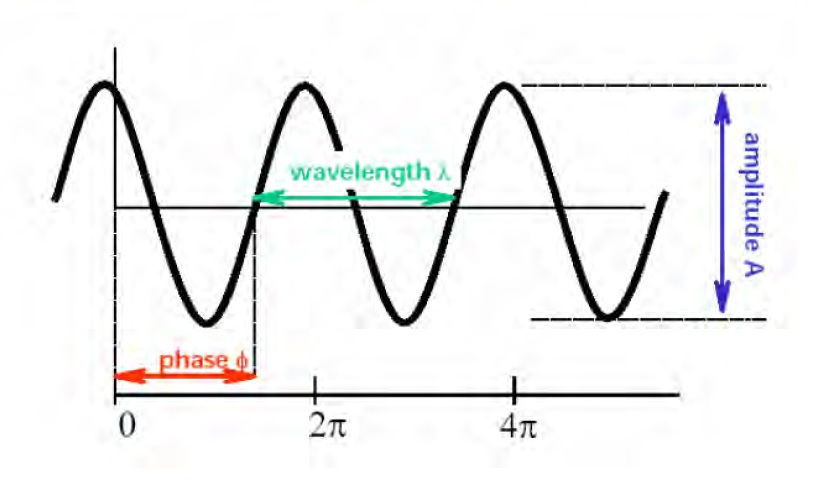
Image 2. Wave Properties Measured by SAR Instruments
From the measured amplitude and phase of a target, we are able to calculate the backscatter intensity (i.e., albedo) and polarization. These are the two main parameters that are measured, analyzed, and quantified in SAR research. The backscatter intensity is a measurement of the number of scattered radar signals that return to the SAR instrument. The greater the signal return, the brighter the radar albedo image. Polarization measures the orientation of the radar signal, and this can provide us with a lot more information about a target than radar albedo. A radar signal is typically orientated in two directions, perpendicular to the plane of incidence (H, Horizontal polarization) or in-line with the plane of incidence (V, Vertical polarization) (see image 3 below). Radar can also be circularly polarized where the direction of the signal is constantly rotating (clockwise or counter-clockwise), but its electric field magnitude remains constant. The course focused on a SAR technique known as dual-polarized radar. This type of SAR only emits and measures H and/or V polarized signals so I will only cover these in this post.
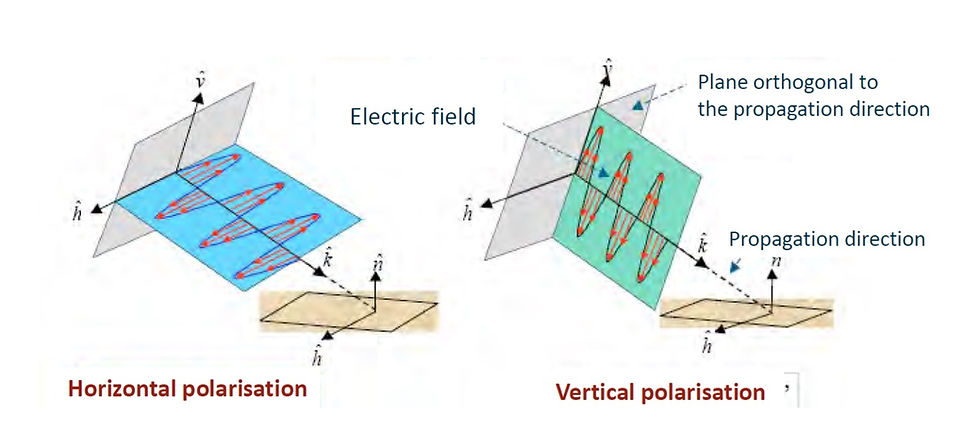
Image 3. Two orthogonal polarization states of radar pulses, horizontal and vertical.
The polarization of a transmitted and returned radar signal can provide us with information about the surface roughness of a target or surface (a physical property I study for my PhD thesis). When a polarized radar signal interacts with a surface and reflects or scatters, it either depolarizes or retains its transmitted polarization (see image 4 below). Depolarization of a radar signal occurs when the transmitted signal interacts with a rough surface. For example, if a SAR instrument emits a V polarized signal and has a strong return of V then the radar signals were not depolarized, therefore the surface was smooth. If the returned signal had a H polarization then the surface is rough (high VH; transmit V and receive H).
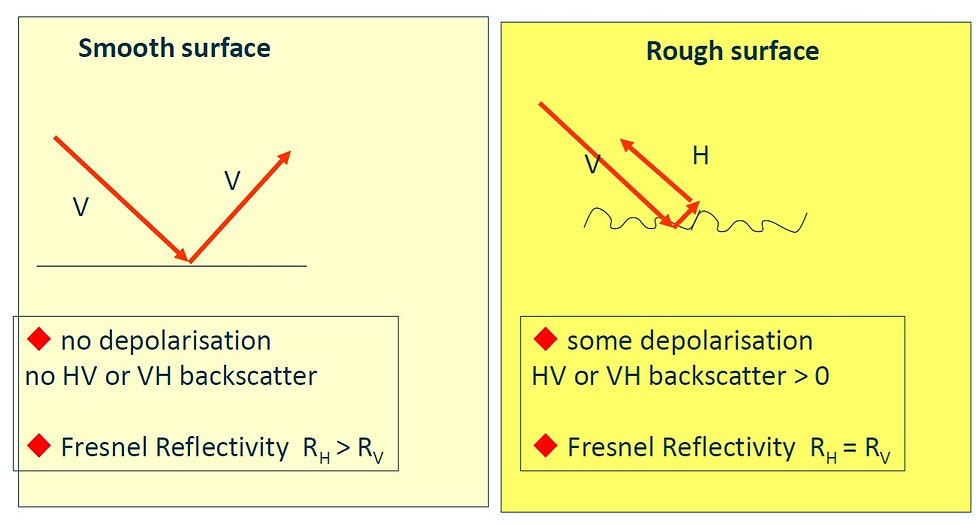
Image 4. Illustration showing how a radar signal either retains its transmitted polarization state or becomes depolarized.
A lot was covered when it came to understanding radar polarization, including the mathematics of calibrating and filtering the data, and understanding the numerous decomposition methods used to process and present the data. We got to learn how polarization of a radar signal changes from different scattering mechanisms (see image 5 below): single-bounce (surface scattering) and volume scattering. Our lectures more on emphasizing that the geometry of a target or surface is never a single shape, but changes across an entire surface and scale (e.g., rough at the centimetre-scale but smooth at the metre-scale; grass). The geometry influences how the radar signals are scattered and how their polarization state changes. We were also taught that the water content of a target will greatly effect the backscatter and polarization of radar signals.

Image 5. Different scattering mechanisms of radar polarimetry.
For me, a lot of the content in the first two days was a recap of what I taught myself during my PhD. Even though it was familiar, it was still good to keep testing myself, to ensure I was ready to talk about SAR during my PhD defense and for conferences and meetings in the future.
Speckle Noise Filtering: An Introduction
This section could go on forever, as there are many concepts to the noise produced by SAR and the methods used to filter the data. I want to mainly focus on giving you a quick introduction to SAR speckle noise and the type of filter I learned about during this course and applied to my thesis.
A SAR image comprises cells, which come from a large number of individual scatterers, each creating a reflected signal with its own amplitude and phase. When the SAR instrument moves over a target, it detects shifting constructive and destructive interference patterns among the individual reflected signals. The variability between the interference patterns are known as speckle noise. The speckle noise in a non-filtered SAR image creates a granular texture, making it challenging to observe specific surface features and interpret the properties of a target. Before analysis of SAR data can commence, the speckle noise needs to be reduced (error of each cell value will decrease). To achieve this, we apply filters that smooth the SAR data. We were taught during the lectures that caution needs to be taken when applying filters, because we need to make sure that we preserve the polarimetric properties, introduce no cross-talk (only a single polarization measurement is made through the antenna and no mixing of measurements occurs), and reduce speckle while preserving image quality.
There are two filters that are commonly used in SAR studies I want to talk about: BoxCar and the Enhanced Lee Filter. Both BoxCar and Lee filter methods are capable of reducing the speckle noise in a SAR image, however, each one alters the image quality and stored data differently (see image 6 below). The BoxCar filter is the most common filter technique used. It replaces the center pixel in a moving neighbourhood (typically 3 x 3 or larger) with an average of the pixels in the neighbourhood. When we increase the filter size (e.g., size of neighbourhood, 3 x 3 or greater), we increase the number of looks in a SAR image. The speckle noise (or standard deviation of the radar echo power) decreases when the number of looks increases. It is worth noting though, that at a certain number of looks, the speckle noise will decrease more slowly.
The advantages of a BoxCar filter includes its simple application, effectiveness to reduce speckle noise in homogenous areas, and being able to preserve the mean value of a pixel. A major disadvantage of the BoxCar filter is its degradation of the spatial resolution of a SAR image. This is caused when pixels in heterogenous areas are averaged with pixels from homogenous areas. Bright points, linear features (e.g., roads, paths, lava flow margins, etc), and edges will be blurred, therefore, the surface features in the SAR image are hidden or worse removed. The Enhanced Lee filter is a more sophisticated version of the standard Lee filtering method and it is able to preserve linear features and edges in SAR images. The algorithm of an Enhanced Lee filter uses a non-square neighbourhood (or window) to match the direction edges. The filter technique uses the local mean and local variance to filter image noise and preserve the image quality and sharp edges.

Image 6. Here is an example of the different filters applied to a 4-look Air Synthetic Aperture Radar (AIRSAR) image of Les Landes Forest. You can see in the boxer (i.e., boxcar) filter image that the speckle noise has been reduced but the edges of the fields have been blurred. In the refined (i.e., enhanced) lee filter image the edges of the fields have been retained. Note: please ignore the MMSE filter image, it is a filtering method I did not learn much about during the course. If you are interested in learning about MMSE filtering I would recommend checking out this site.
SAR Toolbox Applications
On Day 3 of the course, we were given the chance to practice calibrating, processing, and terrain-correcting Sentinel-1 C-band (wavelength = 5.6 cm) dual-polarized radar data using the ESA SeNtinel-1 Application Platform toolbox. In SNAP, we were given instructions on how to convert Level-1 Ground Range Detected (GRD) data into their acquired polarization datasets (e.g., HH and HV). The instructions were pretty self explanatory, and most of the steps I was already familiar with since I use Sentinel-1 data for one of my PhD thesis chapters. What I did not know before taking this course was the ability to batch process images! So many days could have been saved if I knew I could batch process multiple data sets at once instead of going through one data set at a time. We were taught to follow a particular step by step process when working with Sentinel-1 data: Read (SNAP reads the input data) -> Apply-Orbit File (add the orbital position of the satellite when the polarimetric radar data was acquired) -> Subset (crop out a specific region from the image) -> Calibration (convert the amplitude and phase data into the polarization states) -> Speckle Filter (apply a filter to reduce speckle noise) -> Terrain-Correction (correct geometric distortions in the data) -> LinearToFromdB (convert sigma-0 power units to decibels) -> Write (release output data). In SNAP, you can organize these processing steps into a graph builder (see image 7 below), allowing you to visualize the procedure and see what data sets are being put through at each stage. The funny thing is, I knew about the graph builder in SNAP before I started the course. What I didn't know is you can save the graph you developed and input it into a batch process tool, running multiple data sets through each step.
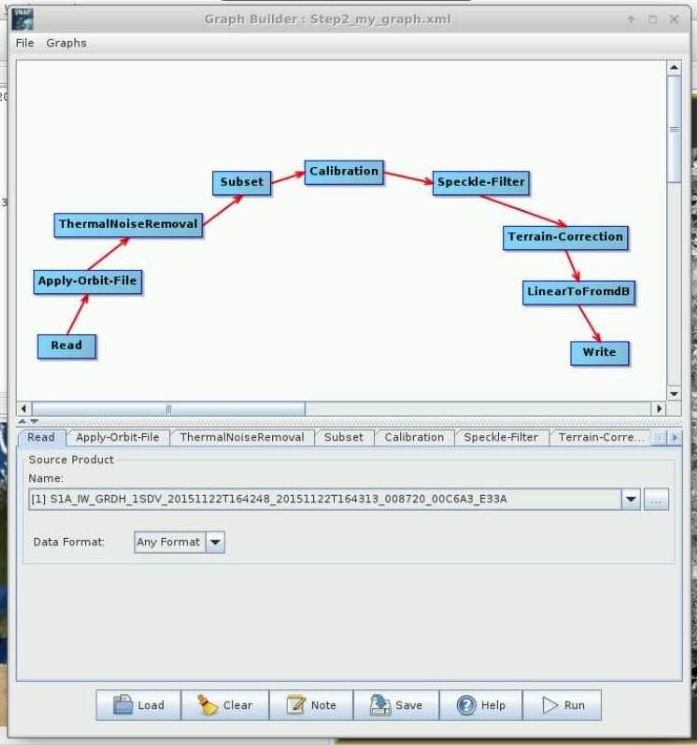
Image 7. An example of a step by step procedure for processing Sentinel-1 radar data in SNAP. The graph builder allows you to visualize each step of the procedure and modify parameters to your desire.
If you work with Sentinel-1 data and are interested in using SNAP, you can download it using the link here. As far as I am aware, the practical's and lectures for the course will be publicly available so you should be able to access the instructions for how to batch process. Until then, there is a short video you can check out that will help you understand how to set up a batch process.
What I Took Away From The Course
I will say the main thing I took away from this course is a greater appreciation for polarimetric radar geoscientists. I knew radar was not a straightforward remote sensing technique, but I never imagined that there was so much more to learn about it, and this post was only a small, tiny fraction of the full content of the course! Something I want to do now in the future is to apply more filtering, processing, and analysis techniques to planetary radar datasets. EO papers use so many techniques to understand the scattering mechanisms, and physical and electrical properties of a surface but only a few are applied to planetary science. Part of this is due to the fact that we have exponentially more terrestrial SAR data than planetary data.
Comments