Surface and Subsurface Radar Scattering
- Gavin Tolometti
- May 8, 2019
- 5 min read
Hello everyone,
It has been an emotional rollercoaster of a month. I made some good progress on my Mistastin research, which has kept me on track but I got some bad news about my Craters of the Moon radar paper. I got an email back from the editor of the journal where I submitted my paper (the editor and journal name will remain nameless). Unfortunately, they were unable to except my paper or recommend it for major edits so it was rejected. That did leave me in a bit of a mood for a while but both of my supervisors Dr Catherine Neish and Dr Gordon Osinski reassured me that everyone (students and professors) experiences rejection when submitting papers to journals. "It does not reflect your ability as a scientist!" (Catherine Neish) What you need to do is learn from it keep on trying :). That really raised my spirit, and it motivated me to read through the reviewers comments and see why the paper was rejected.
So one of the major comments mentioned how do we know the radar backscatter is not a result of buried lava flows or other lithological boundaries? They kept on repeating this throughout the paper. Now, it is not that we didn't know radar could penetrate the surface of lava flows, it was that the dielectric constant of basalt and the 24-cm radar wavelength would make the penetration depth of radar negligible for this research. However, my supervisors and myself thought we should address in case future reviewers raise this question again.
Catherine Neish and myself decided that to prove the backscatter is not a result of subsurface scatter we would calculate the degree of linear polarization (DLP) and see how it compares to the circular polarization ratio (CPR) results already discussed in the paper.
Degree of Linear Polarization
When radar signals interact with the surface of a planetary body they scatter in dependence of the physical and electrical properties, slope, surface roughness, and dielectric constant of the surface and near subsurface. For a quick reminder for any new readers, I have been using radar backscatter to distinguish and quantify the surface roughness of lava flows from Craters of the Moon (COTM) National Monument and Preserve in Idaho. The goal of my research is to observe and understand correlations between the surface roughness, geochemistry, and petrography of the lava flows. The radar surface roughness of the lava flows are presented as CPR. When a radar signal is emitted from an orbiter or low-flying platform it exhibits a specific polarization. Once it interacts with a surface the polarization of the radar signal changes to an opposite polarzation. The CPR value is the ration between the polarization of the emitted signal (transmitted polarzation, SC) and the returned signal (opposite polarization, OC).

Equation from Neish and Carter (2014)
S1 and S4 are two of the stoke parameters (S1 - total average power of the radar echo, S4 - direction and magnitude of the circularly polarized power) that equate the CPR value. For detailed information about these parameters please refer to Neish and Carter (2014).
Low CPR (<0.5) indicates single-bounce scatter, intermediate CPR (0.5- <1) indicates multiple backscatter, and high CPR (>1) indicates a double-bounce backscatter. For a double-bounce backscatter effect to occur on a surface and return CPR values >1, natural corner reflectors, fractures, and cracks must be present. In the field, these features can be wide (>1 m) fractures and cracks in lava flows, cracks in glaciers, and the decimetre to meter-sized blocks on blocky lava flows (e.g. SP Flow in Arizona).
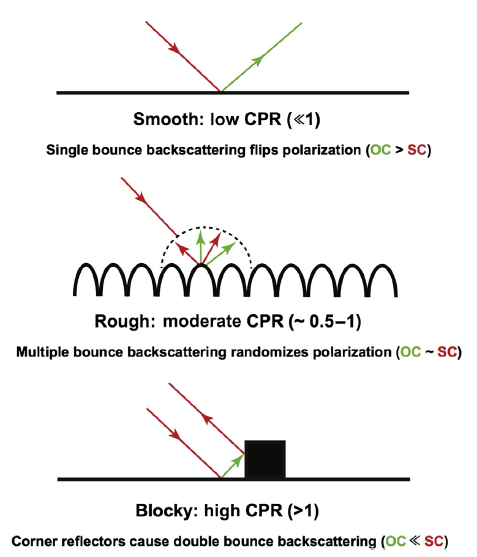
Above figure is from Neish and Carter (2014)
The CPR values of lava flow surface roughness is a powerful dataset to interpret emplacement processes and distinguish different lava facies in remote, inaccessible locations on Earth, and other planetary bodies. However, we must remember that radar signals can also penetrate the surface and interact with subsurface features such as clasts or lithological boundaries (contacts between two different rock units).
In polarimetric SAR images and datasets, scattering caused by the subsurface is calculated as DLP. Using the same stoke parameters used to calculate CPR, we can calculate DLP and quantify subsurface scattering in a surveyed area. The equation below (Neish and Carter, 2014) uses the first, second, and third stoke parameter values, which are the measure of the total average power in the radar echo (S1), and the linear polarized state of the radar wave (S2 and S3). For interest, the fourth stoke parameter (S4) gives the direction and magnitude of the circularly polarized power.
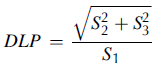
To visualize what DLP values tell us about the subsurface of a study area, think about these examples. If you have a surface with sand overlying a limestone layer then we would have a quasi-specular subsurface scatter returning high DLP values (>0.75). If the surface is rough and beneath it is a different rock layer then the DLP values will be low-moderate (0.1-0.5) as the radar signal may not penetrate through the surface. No rock layers or clasts/buried rocks beneath a surface will return a low DLP value since there is no material to interact with the radar signal. The figure below shows how CPR and DLP vary between smooth and rough surfaces.

Figure is from Neish and Carter (2014)
Craters of the Moon - DLP Results
My supervisor Dr Catherine Neish calculated the DLP values of my COTM study site and produced an ISIS3 cube file I could open and manipulate on ArcGIS 10.5.1. The DLP dataset did not have the correct spatial referencing information and manually inputting the information (taken from Landsat 8 images of COTM) did not seem to work. Instead I had to georeferenced the DLP dataset to a map I had previous made for the research paper. It only took a few minutes to complete (not my first time georeferencing maps or datasets). Before I started any calculations I applied a lowpass filter to the DLP data to reduce the speckle noise effect and DLP standard deviation values. Afterwards, I used polygons I created to quantify the CPR values of my target lava flows and overlaid them onto the DLP data. Using the Zonal Statistics tool in ArcGIS I was able to quantify the DLP of each studied lava flow.
Our results showed that the lava flows I am studying at COTM have a low subsurface backscatter. Their DLP values ranged from 0.15-0.2, indicating the radar was scattered by surface features. From this, I feel more confident in the CPR values I obtained for the research paper and can now add a section to the paper discussing why subsurface scattering is not effecting the radar data.
I do have an image of the DLP results but I want to wait until I have republished my research paper before sharing it online. As soon as the paper is submitted again I will share the figure will you all :)
I reference Neish and Carter (2014) a lot in this blog. Their chapter on Planetary Radar in the Encyclopaedia of the Solar System, 3rd edition, is a very good resource and I would highly recommend it to anyone studying planetary surfaces, radar, and remote sensing applications in planetary science.
See you all next time!
Comments